Micropile Transmission Foundations in Environmentally Sensitive, Challenging Terrain
Abstract
A 13.6 mile portion of the 500 kV Tehachapi Renewable Transmission Project crosses the mountains of the Angeles National Forest. Access and environmental constraints precluded road development for most transmission tower foundations, tower erection and conductor stringing operations. Because of the access constraints’, helicopter-supported micropile foundation construction was used for this steep and environmentally sensitive portion of the project. The general methodologies incorporated on the project represent innovative implementations of micropile design criteria, preconstruction micropile installation and verification testing, on-site geotechnical characterization, micropile and pile cap installation, and proof load testing. Challenges in constructing the 224 foundations for 56 transmission towers provide lessons learned related to transmission lattice steel tower specific design constraints, construction challenges (environmental and geotechnical), and construction schedule.
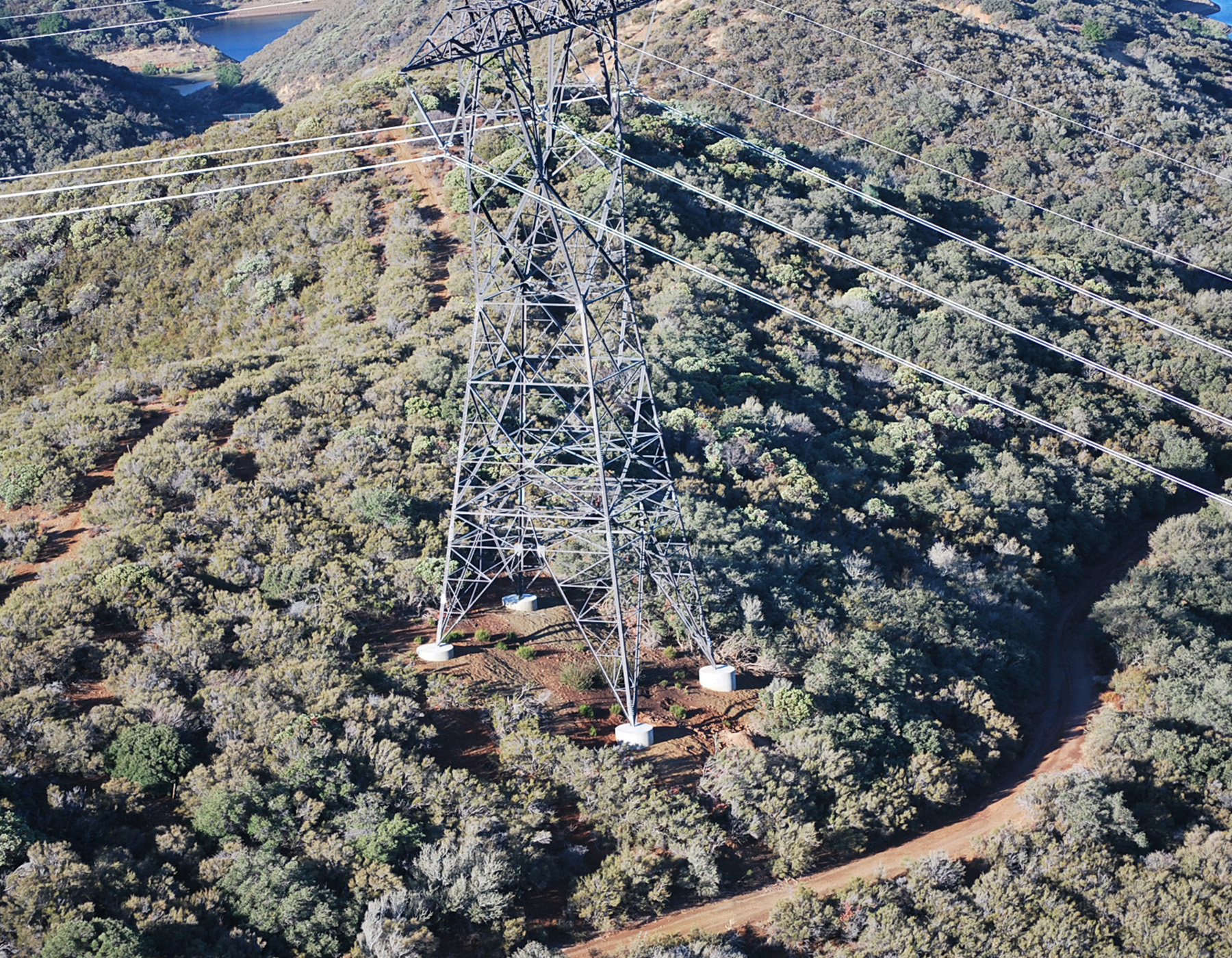
Stress Predictions From Finite Element Modeling
Authors:
Esam Abraham, PE, Southern California Edison, Rosemead, CA USA, Chih-Hung Chen, PE, Burns & McDonnell, Kansas City, MO USA, Pete L. Hlapcich PE, Southern California Edison, Rosemead, CA USA, Donald E. Johnson, TRTP PM, Southern California Edison, Rosemead, CA USA Norman W. Jufer, PE, Southern California Edison, Rosemead, CA USA, Nickolas G. Salisbury, President, Crux Subsurface, Inc., Spokane Valley, WA USA Hannling Shaw, PE, Southern California Edison, Rosemead, CA USA
Introduction
Southern California Edison (SCE) is a California utility working to meet state regulations mandating that 20 percent of delivered power come from renewable sources by 2010. SCE is expanding its transmission system to bring generation from wind farms in and around the Tehachapi Mountains in Kern County into Los Angeles. Conventional drilled piers are the typical foundation systems used for lattice transmission towers. These require roads for drilling rigs and concrete trucks during construction. However, for a 13.6-mile stretch of 26.5-mile Segment 1 of the Tehachapi Renewable Transmission Project (TRTP), unconventional foundation construction methods were used to accommodate tower sites without access roads. This section crosses the remote mountains of the Angeles National Forest (ANF), as shown in Figure 1.
Micropiles were conceived in Italy in the early 1950's for underpinning historic buildings and monuments that had sustained damage over time. The micropile systems used today have evolved from basic small-diameter, cast-in-place piles. Used for more than 20 years in the U.S., micropiles are widely used in supporting buildings, bridge piers, earth retention systems, and for landscape stabilization. This foundation technique has been used for about a decade to support electrical transmission structures, although this may be the first successful use on four legged lattice transmission towers (see Figure 2).
It was a logical choice for the sensitive environment, confined working space, and variable geological conditions on this project. Helicopters played a major role during construction of the foundations, erection of lattice steel towers, and for wire stringing, where the 500-kV overhead line crossed U.S. Forest Service (USFS) lands.
Environmental Restriction
The California Environmental Quality Act (CEQA) is administered by the California Public Utilities Commission (CPUC) as it relates to utility work. It requires SCE and its contractors to implement specific environmental mitigation measures adopted during the permitting phase of the project in order to begin construction. The ANF is home to many protected plant species and is the natural habitat of endangered California condors and migratory bird species.
Because of this sensitive habitat, no new access roads were allowed to be constructed, except as necessary and temporary for wire stringing. Disturbed areas had to be minimized, and work was required to slop in the presence of active bird nests or protected species. Without an access road to move drilling equipment and cranes to tower sites, a conventional drilled pier foundation would require hand digging. Spoils would have to be hauled out by helicopters.
Small-profile drilling rigs could be transported to sites by heavy lift helicopter, but the USFS did not allow the major grading to level tower sites required for their use. The use of explosives to excavate boreholes was not permitted because it could potentially increase the danger of fire and impact wildlife. Micropile foundations presented solutions to this unique combination of obstacles (see Figure 3).
The USFS, upon CPUC approval of the project, issued a Special Use Permit (SUP) that ordered detailed mitigation, monitoring, and compliance plans be in place in order to begin construction. Helicopter-supported micropile foundation is one of the SUP-approved construction methods proposed by SCE and its contractor. This method minimized the environmental impact by taking advantage of a smaller foundation construction footprint, eliminating the need for road building, and reducing spoils and air emissions compared with conventional drilled pier construction.
Geology
TRTP Segment 1, Section 2 includes structures 29 through 88 and is located in the Sierra Pelona area of the ANF in northern Los Angeles County. Bedrock encountered along the alignment includes the Miocene Mint Canyon Formation (Tmc), Mesozoic quartz diorite (qd) and Mesozoic or older Pelona Schist (ps) as shown in Figure 4.
The Mint Canyon Formation unconformably overlies the Pelona Schist in the southern portion of the segment (Structures 29 through 33). The Mint Canyon Formation consists of interbedded non-marine clastic sediments including claystone, siltstone, sandstone and conglomerate. The unit is typically thick bedded with well defined bedding in the siltstone and claystone units. This unit is a relatively soft sedimentary rock unit where unsupported, slope instability can result as documented by the landslides mapped in this area.
Most of the structures in the segment are underlain by the Pelona Schist (Structures 34 through 74). The Pelona Schist is exposed in the central portion of the Sierra Pelona and consists of a mica-quartz-albite-chlorite schist derived from marine deep-water sand, silt, calcareous and siliceous mud and sparse basaltic tuffs and flows. The Pelona Schist displays well developed foliation and joints. The Pelona Schist is a crystalline bedrock unit and is generally very hard, where unweathered. Because of its clay composition, however, the Pelona Schist weathers readily resulting in soft clay zones that can create potential slope instability. The Clearwater Fault defines the contact between the Pelona Schist and quartz diorite to the north (Structures 75 through 88).
Quartz diorite consists of gray medium grained diorite composed predominantly of plagioclase feldspar and quartz with minor biotite and hornblende. Quartz diorite is a crystalline bedrock and is generally massive and hard.
Foundation Design
The lattice-type transmission tower foundation design had to consider the following factors:
- Design loads on the foundations
- Settlement and deflection criteria
- Stub angle installation tolerances
- Grounding detail requirements
- Geotechnical recommendations
- Environmental requirements
- Schedule requirements
- Cost
A proper foundation system can be developed for a specific project by taking account of these factors. The most common foundations for lattice-type transmission towers are cast-indrilled-hole (CIDH) pile footings. This type of foundation is also known as drilled pier foundations. The design of a typical drilled pier foundation involves the determination of diameters, lengths, concrete strength, reinforcements, etc., to resist the loads imposed by the lattice transmission tower within the foundation material capacities.
Typical drilled piers for lattice steel transmission towers would be 3.5 to 6 feet in diameter and 15 to 30 feet or more in depth. A structural angle is embedded in the pier concrete and used for attachment of the tower leg angles to the foundations. These angles are known as stub angles and require precise location.
Micropile construction eliminated the necessity of hand digging the foundations within the ANF and a variety of issues associated with this. In addition, micropiles are a proven technology with wide application. In considering the design of micropiles for this use, SCE's main concerns were:
- The ability to maintain tolerances for stub angle setting.
- Keeping foundation deflections within acceptable limits.
- Preventing corrosion and deterioration of the above ground portion.
- Securing any adjustment mechanism for alignment to avoid movement after tower setting.
The first designs considered involved a direct connection of the micropile or micropiles to an adjustable steel base plate to which a stub angle attachment would be welded. For medium-duty tangent towers, a single pile foundation was proposed that involved a coupler welded to a steel adapter plate on top of the micropile. This adaptor plate was designed to interface with the base plate by means of three slotted holes. Small adjustments could be made vertically, rotationally, and translationally. Similar designs were considered with a minimum group of three battered micropiles. Concerns with these designs were deflection under load, limited adjustability of the stub angle to construction tolerances, the need for a high level of quality control for welding, and corrosion potential for the exposed parts of the pile group cap.
In order to assure the structural integrity of its lattice towers, SCE required that the lateral foundation deflection at the top of the pile cap under working loads be limited to a maximum of 3/8 Inch. This requirement and the above mentioned concerns led to the use of a concrete pile cap, shown in Figures 5 and 6, as the preferred design. With this solution, the stub angle setting and micropile terminations are separated, allowing workers to set the stub angles in a traditional manner. The concrete pile cap provides the necessary rigidity to prevent excessive deflections while mobilizing the strength of the micropile group to support the transmission tower. The concrete pile cap in a typical installation can be kept within 5 feet to 6 feet in height while meeting bonding and punching shear requirements and SCE's requirement for some minimum concrete embedment in soils in case of erosion. The resulting pile caps had a desired low profile to minimize excavation. The reinforcing required for this design resulted in a crowded but workable steel layout.
The concrete pile cap was designed in accordance with ACI 318-95 and CRSI Design Handbook. The depth of the pile cap is determined by the embedment depth of tower stub angles and the punching shear requirements. The factored bearing, uplift, and shear loads are used to design flexural reinforcements and embedment depths for punching shear or pullout. The compressive strength of concrete and yield strength of steel reinforcements are 3,000 psi and 60,000 psi in the pile cap design.
Micropile Design Methodology
The micropile foundations for this project were designed using a varying number of micropiles installed in a circular array. The micropiles are battered away from the center and derive their capacity from the native soils/bedrock at each site. The proposed micropile groups were analyzed using GROUP 7.0 (GROUP) software developed by Ensoft, Inc. GROUP analyzes pile groups subjected to vertical, lateral and overturning loads in a two-dimensional model for symmetric pile groups, or in a three-dimensional model for symmetric and asymmetric pile groups. In these analyses, a three-dimensional pile group configuration with fixed head conditions has been modeled. GROUP requires input values that include foundation loading, pile coordinates and orientation, pile properties, geotechnical profile, and geotechnical properties. GROUP does not accommodate modeling of groups of piles on a slope. A specific methodology was developed to assimilate a reduction of near-surface soil and rock support due to sloping ground. Outputs from GROUP are used to determine the depth of fixity (zero moment), foundation deflection, pile moment loading, and stress along the length of the pile as well as the individual pile axial load.
An individual micropile resists the lateral loads and associated moments applied to the group by transferring a portion of the load into axial (due to the batter) and resisting the remaining portion by the interaction of the bending strength of the pile and the strength of the geotechnical unit. The depth of the upper cased section of the micropile is determined by the moment curve, as developed by GROUP. Appropriate casing embedment depths for each pile are noted on the foundation schedule issued for construction. For design simplification to account for ground surface potential erosion, analyses were performed using three "idealized" subsurface conditions and likely steepest slopes associated with these soil conditions.
Micropile capacities are designed in accordance with the Federal Highway Administration {FHWA) "Micropile Design and Construction Guidelines" {FHWA-SA-97-070). The axial capacity of a pile is developed in the bond zone section of the pile {lower uncased section) as shown in Figure 7. One design criteria used in the layout of the micropiles was a requirement that each bond zone be separated by a minimum of 30 inches, eliminating any negative axial group effect as defined by FHWA. Soil strength values for design of the bond zones were obtained from the Post-Tensioning Institute (PTI) "Recommendations for Prestressed Rock and Soil Anchors" (ISBN 1-931 085-29-3). Ultimate tests have modified or verified ultimate grout-to-ground bond stresses for each of the geotechnical units. Appropriate bond lengths for each pile are shown in the Foundation Schedule. The maximum axial uplift of individual piles was evaluated for cone pull-out. Cone pull-out did not control elements of the design.
Preproduction Verification Tests
Preliminary design documents were completed using generic ultimate grout-to-ground bond stresses from the FHWA manual and the PTI manual for each of the three geologic bedrock units and for prevalent soil conditions identified along the alignment. Ultimate Tests were recommended to confirm or modify the values used in the preliminary design for inclusion into the final design documents. (See Figure 8) The materials used in the preproduction tests may not be the same materials planned for the permanent micropiles on the project, but it was sufficient to achieve the predicted failure of bond. Tension tests were conducted rather than compression to evaluate the grout to ground bond excluding any contribution from end bearing. Lateral tests were not conducted on individual piles due to the inapplicability to groups of piles with opposed batters, Instead, computer models were conducted for groups of battered micropiles in known soil conditions. Bond breakers were installed in the unbonded portion of the pile, using a PVC sheath surrounded by bentonite (weak) grout. No permanent casing was necessary nor installed in the upper section of the pile.
Four locations with two micropiles at each location were initially selected along the alignment to facilitate the use of the existing forest road system. A representative subsurface location within each distinct geologic unit was chosen for the ultimate tension tests. (See Table 1) Where many rock masses typically exhibit a decrease in weathering and gain strength with depth, the pelona schist remained highly variable in quality throughout the mass. After the pelona schist rock tests, a second location was added to provide additional data for a comparison in this unit. The lowest calculated bond stress for each unit was chosen as the final design value.
Foundation Construction
The construction team faced the challenge of planning construction activities to accommodate the daily Project Activity Level (PAL) issued by the USFS. This system is implemented to minimize the risk of fire in the forest. The rapidly changing weather and wind condition in the mountain elevations ranging from 2,000 feet to 4,500 feet brought challenges to the helicopter operation. On numerous occasions, work ceased due to snow, fog, hail, or high wind. All field personnel were trained and equipped to handle inclement weather, and helicopter pilots were on-call to evacuate personnel when weather risks were identified. On several occasions, crews were required to hike from remote tower locations to adjacent roads when helicopter transportation was not an option.
A total of 56 towers (224 foundations) in the national forest were constructed using micropile foundations with cast-in-place concrete pile caps. Foundations for tangent-type towers were constructed with composite micropiles consisting of a 5.50-inch outside diameter (OD) upper cased section, and a 1.375-inch diameter 150 ksi Williams All-Thread rebar. Foundations for angle- and deadend-type towers were constructed using an 8.625-inch OD upper cased section and 2.50-inch diameter 150 ksi Williams All-Thread rebar. The number of micropiles in each tower leg ranged from 3 to 12. Pile lengths varied from 25 to 51 feet. Approximately 1,000 micropiles were installed with a combined lineal footage of 30,000 feet.
The micropile drilling, grouting, and testing equipment and other materials were designed and ordered to be within the helicopter lifting capacity as well as to enhance the foundation construction productivities. The construction activities followed the sequence described below:
Excavation of Pile Caps — The height of the pile cap was controlled by the length of the tower stub angles. Because of this and an allowance for erosion of soil cover, it was necessary to embed part of the pile cap below the existing ground elevation. The depth and diameter of each excavation was controlled by leg-specific elevations, tower leg extensions, and micropile foundation design. Cap excavations were accomplished by hand, using air tools where necessary. Spoils were side cast on site and used as backfill around the caps upon completion.
Micropile Installation — Specially designed and manufactured drill platforms and componentized drill rigs were flown in and set up over the open pile cap excavation at the leg of the tower site. (See Figure 9) High pressure/volume air compressors were flown and positioned within the tower footprint that could accommodate construction of all four legs, eliminating repositioning. A grout plant or grout transfer unit was flown to a central area at each site, as were tool boxes, personnel work platforms, firefighting equipment, and installation materials. All equipment was custom designed to be securely leveled on steep slopes without the need for benched excavations. In addition, all rotary machines are Environmental Protection Agency (EPA) rated environmental friendly and were inspected and tested by the USFS fire marshal as part of SUP requirements.
Standard Penetration Tests (SPT) were performed at specific elevations to characterize the soil conditions while drilling the first micropile at each tower site. SPT blow counts (N) were input into the Foundations Schedule which acts as a decision matrix for properly constructing the foundation to match tower loading and soil conditions. The Foundation Schedule provides guidance in determining the number of piles necessary, the length of casing embedment, the bond length, and grouting method to be used in the installation of each pile. This design and installation method provides assurance that the foundation will generate the necessary capacity without installation of additional costly and unnecessary capacity, often inherent in the design of more conventional foundation systems. This type of Foundation Schedule is currently pending patent approval.
The drill platform, drill rig, and materials are moved to each subsequent leg at the tower site using the same decision matrix continuing pile installations. Grouting for Type B micropiles (pressure grouted piles) was performed with the drill rig remaining on the tower leg foundation, while many Type A micropiles (gravity grouted) were grouted after all micropiles at a tower site were drilled.
Proof Test - This testing program is similar to the Ultimate Test. Figure 10 shows the test beam setup in an inclined angle to be perpendicular to the battered micropile in order to perform proof test. A minimum of four days was required to cure grouts before proof load testing of the micropile. A minimum of one micropile was tested at each tower site and was loaded to the maximum factored design micropile load for that tower foundation. (See Figure 11)
Micropile foundation installation took place from December 6, 2008 through May 18, 2009. On average, without work restrictions, each drill rig and supporting crews completed a tangent tower leg per 10-hour shift. Four drill crews were used for the majority of the project, so the progress of micropile installation for the tangent tower foundations was constructed an average one tower per day. As for the deadend and angle towers, the average progress is about two days to complete micropile installation per crew.
Pile Cap Installation - After proof load testing, micropiles were trimmed to meet vertical tolerance as specified and bearing plates were installed. Crews followed behind to install and tie pile cap rebar, installing grounding as specified and erecting concrete forms around the pile cap. Stub angles were set with precision to meet specified tolerance; the concrete was cast, the forms stripped, and backfill was placed to prevent erosion around the completed cap.
One challenge of helicopter-supported foundation construction lies in the transportation of grout and concrete. Once grouting of micropiles or casting of a pile cap begins, the transportation of that material must be prioritized over other activities. The continuous placement of grout or concrete is necessary to maintain the integrity of the structural element. Because of the PAL and changing daily weather conditions, it was difficult to manage and plan construction activities. On site volumetric concrete complying with ACI 304.6R-09 guidelines was used for batching concrete. Its advantages over batch plant concrete include consistent production of small volumes of close-tolerance, high-quality concrete when needed to transport by medium lift helicopter as shown in Figure 12.
The average volume of the four pile caps varied from 10 cubic yards to 36 cubic yards for the tangent and deadend tower foundations, respectively. The average daily progress ranged from two towers for the tangent type structures to one tower for the angle and deadend type structures without work restrictions.
Conclusion
The Angeles National Forest section of TRTP features the first lattice steel towers in SCE's transmission system to use micropile foundations. The installation successfully met project engineering and regulatory requirements. Based on TRTP's limited road access and disturbance requirements in the ANF micropile foundations for lattice steel towers provided a viable option to standard drilled pier foundations. Concrete pile caps were used on this project and provided the necessary structural stiffness and strength to support the transmission towers. The concrete caps also provided for the separation of pile terminations from tower stub angle setting. This allows for the setting of tower stubs angles through traditional means and within required tolerances.
Reference
AMERICAN CONCRETE INSTITUTE COMMITTEE 304, 2009. Guide for Use of Volumetric-Measuring and Continuous-Mixing Concrete Equipment (ACI 304.6R-09). American Concrete Institute, Farmington Hills, Mich.
AMERICAN CONCRETE INSTITUTE COMMITTEE 318, 1995, Building Code Requirements for Structural Concrete (ACI 318-95) and Commentary (ACI 318R-95), American Concrete Institute, Farmington Hills, Mich.
ARMOUR, T., GRONECK, P., KEELEY, J., and SHARMA S., 2000, Micropile Design and Construction Guidelines Implementation Manual. Report Number FHWA-SA-97-070, Federal Highway Administration Region 10.
CONCRETE REINFORCING STEEL INSTITUTE, 1996, CRSI Design Handbook, Concrete Reinforcing Steel Institute, Schaumburng, IL.
ENSOFT, INC., 2006, GROUP 7.0, Analysis of a Group of Piles Subjected to Axial and Lateral Loading (Computer Program), Ensoft, Inc., Austin, TX.
Geotechnical Engineering Report, Tehachapi Renewable Transmission Project (TRTP), Segment 1, Section 2, Lancaster Vicinity, Los Angeles County, California, Terracon Consultants, Inc., Irvine, CA.
POST-TENSIONING INSTITUTE, 2004. Recommendations for Prestressed Rock and Soil Anchors, PTI
Authors:
Esam Abraham, PE, Southern California Edison, Rosemead, CA USA, Chih-Hung Chen, PE, Burns & McDonnell, Kansas City, MO USA, Pete L. Hlapcich PE, Southern California Edison, Rosemead, CA USA, Donald E. Johnson, TRTP PM, Southern California Edison, Rosemead, CA USA Norman W. Jufer, PE, Southern California Edison, Rosemead, CA USA, Nickolas G. Salisbury, President, Crux Subsurface, Inc., Spokane Valley, WA USA Hannling Shaw, PE, Southern California Edison, Rosemead, CA USA
Published By: Deep Foundations Institute, August 2, 2010